Undoubtedly, you’ve heard the term GPS. It’s not uncommon for consumers to refer to navigation software like Waze, Google Maps, Apple Maps or a portable navigation device from Garmin or TomTom as a GPS. Unfortunately, giving these solutions the GPS title is incorrect. Let’s look at GPS, how it works, and how similar technologies are evolving.
What Is GPS?
The Global Positioning System (GPS) is one type of global navigation satellite system (GNSS). A GNSS uses an array (also called a constellation) of satellites that broadcast extremely accurate time signals. A receiver on the ground (or in your vehicle, a portable navigation device or a smartphone) receives several of these signals. Based on the information from multiple satellites, the receiver can calculate its location and altitude.
GPS was a project started in the early 1970s by the U.S. Department of Defense to provide accurate location information to military troops and was initially called Navstar GPS. Previous terrestrial locating systems such as Decca, LORAN and Omega used longwave radio transmissions from ground-based antennae. A receiver would capture a master signal, then signals from slave transmitters. Analyzing the time differences between the signals allowed for location calculation.
GPS uses a satellite constellation that circles the globe in medium Earth orbits about 20,000 kilometers above the ground. The first satellite was launched in 1978, and the full constellation was completed in 1993. There are currently 32 operational satellites, and the United States Space Force operates the system.
How Does GPS Locating Work?
Each GPS satellite orbits the Earth once every 11 hours, 58 minutes and 2 seconds. Each satellite continuously transmits a signal containing the satellite’s orbit details and precise time information from an onboard atomic clock. The receiver can calculate the distance to the satellite by comparing the time that the signal left the satellite to when it arrived at the antenna. Once the receiver has distance information from several satellites, it can attempt to pinpoint a location using a process called trilateration.
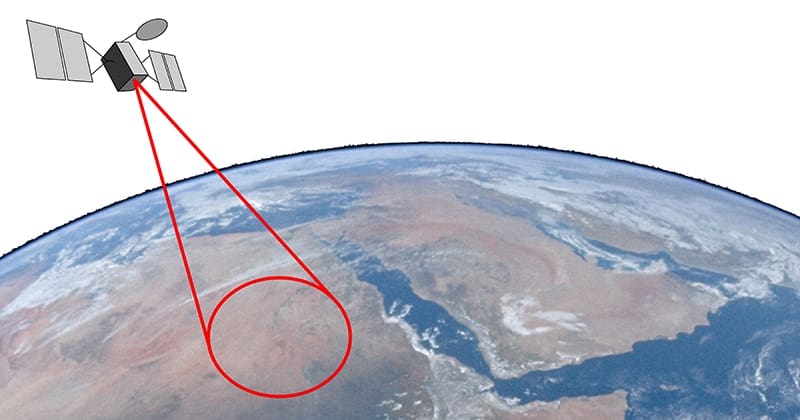
In the image above, we see a circle on the Earth. This circle represents all the possible locations where the distance between the ground and the satellite is equal. Let’s say the satellite is 26,000 kilometers away from this location. It would take 0.0867266647515195 second for the signal from the satellite to reach any place on this red circle. This information alone doesn’t tell us much about the location, other than perhaps what part of the planet we are on. We likely already know that. Let’s add another satellite.
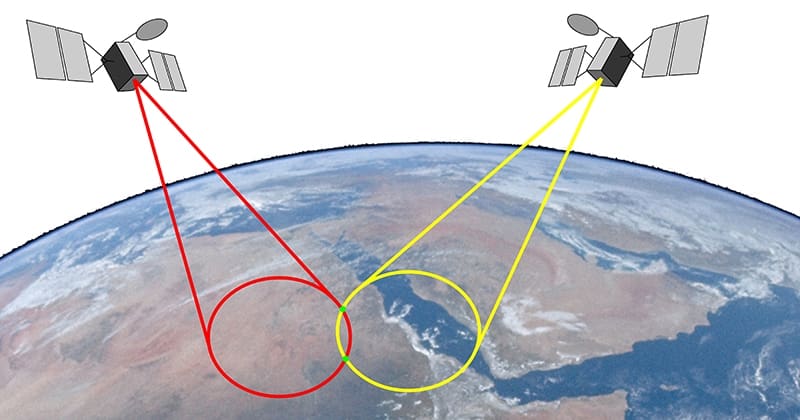
We’ve added information from a second satellite, shown by the yellow circle. This satellite appears to be a bit farther away from our location at 29,000 kilometers. It would take the signal from the satellite 0.0967335876074641 second to travel that distance.
Whatever GNSS receiver we are using can analyze the data from the satellite and determine that we are 26,000 kilometers from one source and 29,000 kilometers from the other. This information gives us two possible locations on the Earth’s surface. The small green dots in the image above mark these locations. We are close to having our position, but we need more data. One more satellite is required.
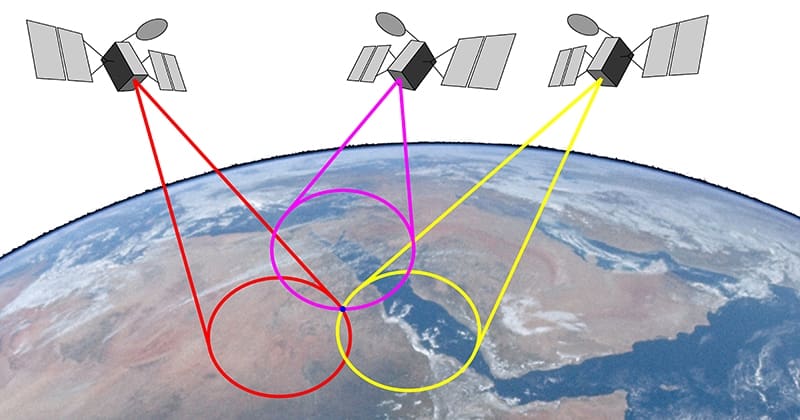
We’ve added a third circle. This satellite might be 22,000 kilometers away. As such, we know the time it takes for the data to arrive at our receiver should be 0.0733841009435934 second. Only one location on the Earth has these three signal travel times to their respective satellites. The blue dot represents that location.
Satellite-Based Positioning System Accuracy
Unfortunately, the clock on our GPS receiver is nowhere near as accurate as the atomic clocks on the $250 million satellites. This reduction in timing accuracy decreases the location accuracy as we have to throw away some of those decimal places from the timing calculations. Some quick math shows us that limiting the timing accuracy to eight decimal places reduces the accuracy to an area of over 2 meters when the satellite is far away and about 30 centimeters when the satellite is close.
Other issues like atmospheric effects can cause timing errors. Part of the cost of operating GPS is constantly checking and calibrating each satellite’s signals. High-precision receivers at fixed locations on the ground continuously monitor the signals from all the orbiting satellites. Measurement of errors because of humidity, atmospheric pressure and ionospheric delay can reduce accuracy to dozens of kilometers. The operators use data from fixed ground stations to create compensation signals transmitted back to the satellites to counteract these and many other errors.
In real-world applications, GNSS systems require data from at least four satellites, as this allows for some error correction. Consumer-level GPS receivers typically have a published accuracy of about 30 centimeters or 1 foot. The latest high-precision receivers can provide locations with an accuracy of 8 mm in longitude and latitude and 15 millimeters in altitude.
If you’ve seen a YouTube video of a 3D drone show, they use GNSS modules that provide location accuracy with real-time precision of about 1 inch.
Below, a fleet of 200 Lumenier Arora light show drones doing testing at the Freedom Factory in Florida.
Are There Alternatives to GPS Locating?
While everyone talks about GPS locating as the de facto standard in GNSS systems, the reality is that many similar systems are in operation around the world. Aside from the U.S.-operated GPS, the Russian Federation operates GLONASS, China has the BeiDou system, and the European Union has Galileo. There are also regional systems like India’s NavIC and Japan’s QZSS. As of this article’s writing, there are 136 GNSS satellites in operation around the Earth, with 15 more as backups or in various commissioning stages.
The highest precision receivers, like those used for military and surveying, can simultaneously receive location data from multiple systems to increase accuracy. Many solutions, like Galileo, offer precision down to 1 centimeter or 0.39 inch with access to encoded data.
How Do We Use GNSS Services?
Navigation systems are among North America’s most popular consumer applications for GNSS information. Whether you have a portable navigation device (PND) like a Garmin or TomTom or rely on a smartphone-integration solution like Google Maps, Apple Maps or Waze, these systems pinpoint your location on a map database using a combination of GPS and location calculation using a system called Assisted GNSS. If you’ve ever put an iPhone into airplane mode, you may have seen a message about location services being more accurate when connected to a cellular service. Assisted GNSS downloads position data from a server over the cellular data network. In short, this technology knows which cellular towers you are close to and can use that information to help triangulate your location. Data about which GNSS satellites are in (relatively) close proximity is also downloaded to speed up the acquisition time of the GNSS receiver.
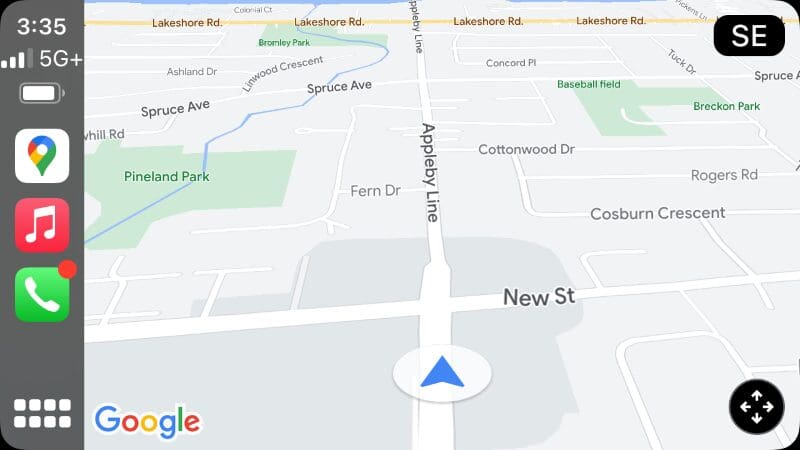
Of course, tracking and locating systems like the popular DroneMobile solution from Firstech combine a GNSS receiver with a cellular data radio. You can control a Compustar remote car starter or security system from the DroneMobile app on your smartphone. If you’ve signed up for location-based services, you can pinpoint the position of your vehicle or configure location-based alerts to help you monitor your car or truck.
GPS location information and tracking are great for hikers who want to venture into the woods and still find their way back home. Devices like SPOT satellite communication devices use GNSS information with Globalstar satellite messaging services to provide emergency support services almost anywhere on the planet.
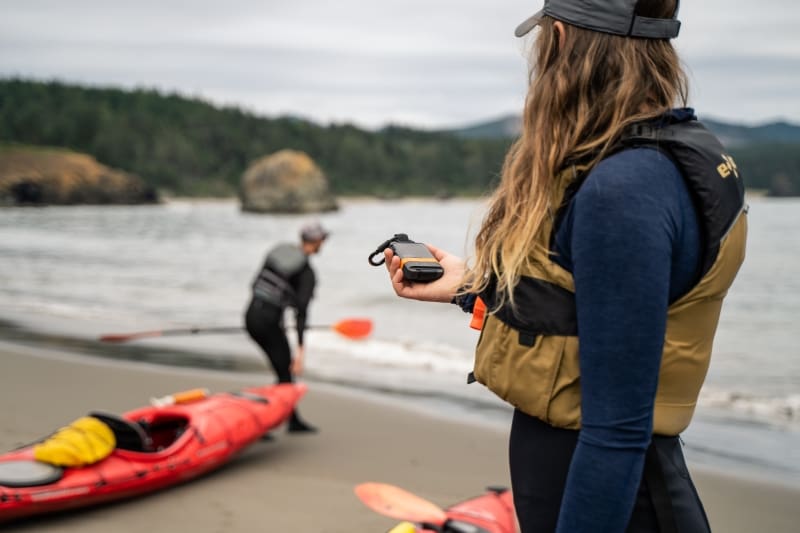
There are dozens of commercial applications for GNSS data. Construction of large buildings often starts with surveying. Precision survey equipment uses GNSS data to mark property boundaries and denote parking lot elevations for proper drainage. The corners of building footings are also crucial identifiers based on GNSS data. If you’ve ever wondered how bridges are constructed simultaneously from opposing sides of a river to meet perfectly in the middle, it is attributable to accurate GNSS data. Modern construction equipment also uses GNSS data to provide exact elevation information.
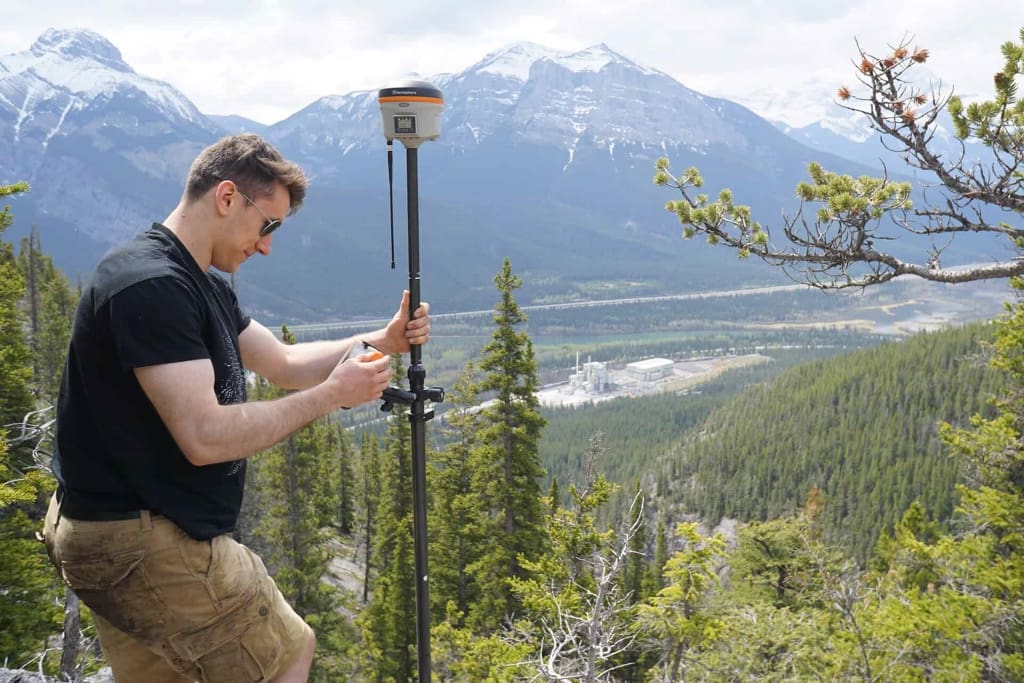
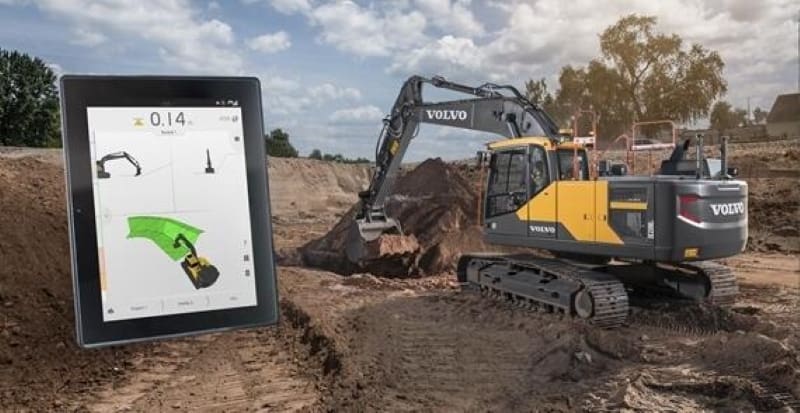
Almost every commercial vehicle has location tracking — from airplanes and ships to trains, buses and transport trucks. Companies must keep track of their assets and ensure that they are safe and comply with company usage guidelines. GNSS information can help global operations avoid weather concerns.
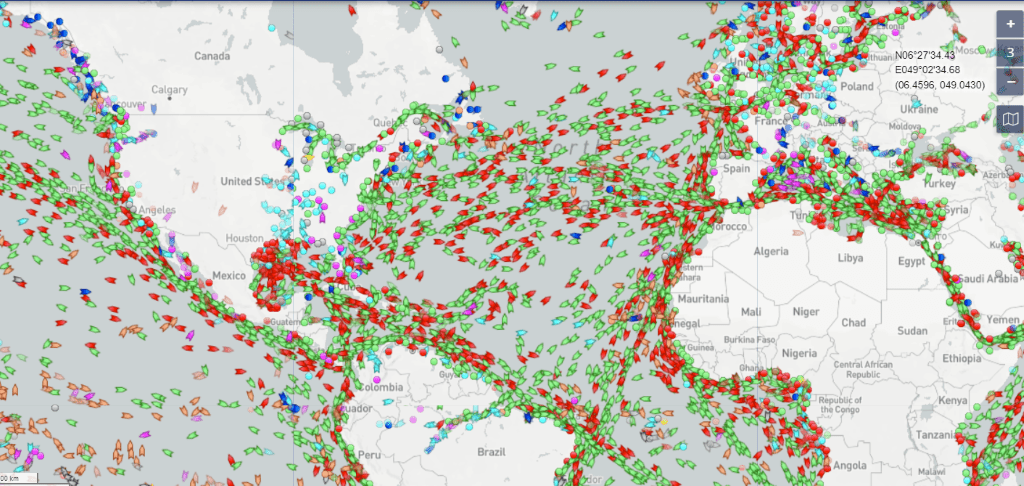
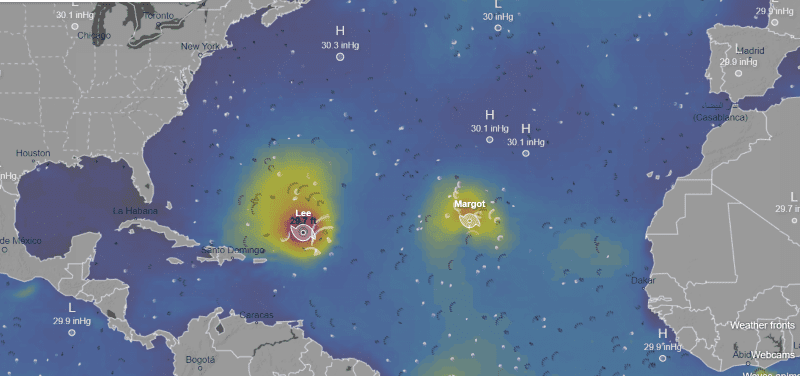
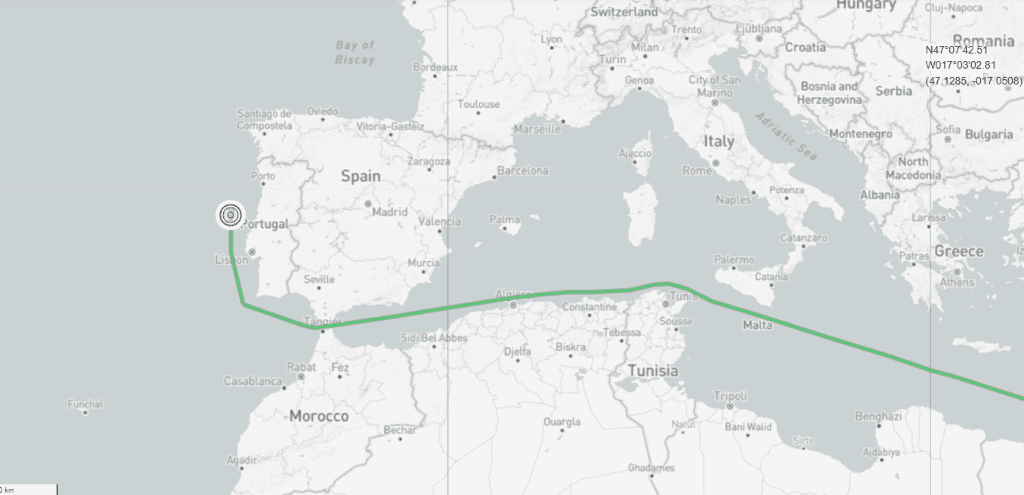
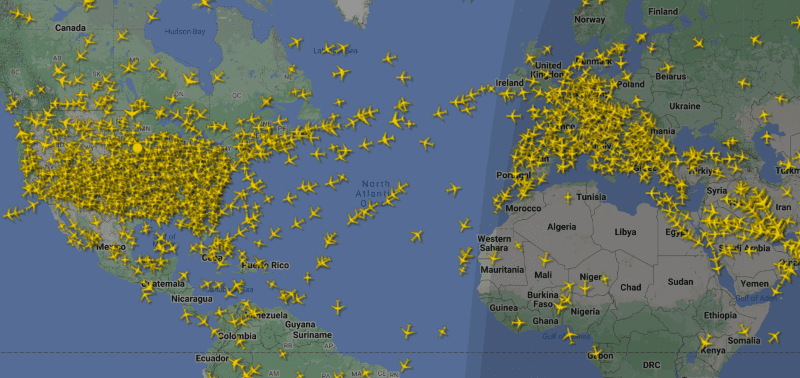
The Future of GNSS Information
So what does the future hold for location information services like GPS, GLONASS and BeiDou? Companies like ALPS Alpine are working on location-based sensors for vehicles as part of the vehicle-to-everything (V2X) push. These products will dramatically improve autonomous driving technologies and help develop intelligent AI-based accident prevention as vehicles can communicate with one another with impressive location precision.
For now, when someone tells you to use GPS to get directions, they are referring to a navigation device that uses some or many forms of GNSS, including GPS locating. If you want to integrate the latest navigation technologies into your vehicle, drop by a local specialty mobile enhancement retailer and ask about a new radio or smartphone integration solution with Apple CarPlay and Android Auto.